CAREER: Electrochemically Active Biofilms (NSF #0954186)
Electrochemically active biofilms (ECABs) where microorganisms are grown through the direct electrochemical stimulation via electrodes, as opposed to being exposed to chemicals that serve as electron donors and acceptors, are widely used in bioremediation, wastewater treatment, biofuel and biochemical production, microbial fuel cells, corrosion studies, and in sensor development. The electron transfer processes between biofilm cells and the solid electrodes, however, are poorly understood. This research quantifies redox-mediated electron transfer rates with the goal of determining fundamental scientific understandings of how to stimulate or, depending on the application, limit electrochemically-induced cell growth rates and/or product formation. The work will also create a quantitative, mechanism-based, mathematical model that can be used to predict rates of biofilm cell growth and product formation using electrochemical, diffusion-reaction, and genome-scale constraint-based models to predict electron transfer rates and their impact on microbial metabolic processes. Broader impacts of the work are far-reaching and include potential societal and economic implications related to microbial fuel cells, biofuels, bioremediation, and biomedical applications. In addition, the project demonstrates the strong integration of education and research that includes creation of a novel, hands-on, microbial fuel cell education module for college undergraduates and another for high school students. High school teachers and students from schools in Idaho and Washington State with high Hispanic and Native American enrollments will be engaged in the project to try and broaden participation of minority students in STEM fields.
Electron transfer mechanism in biofilms (ONR)
Although interactions of microorganisms with electrodes have been studied in the literature, the mechanisms of electron transfer are still poorly understood. The goal of this proposal is to investigate electron transfer mechanisms in biofilms growing on electrode surfaces acting as anodes or cathodes using microelectrodes, nuclear magnetic resonance (NMR) spectroscopy, and imaging techniques. The proposed project quantifies chemical and electrochemical gradients in anodic and cathodic biofilms. We demonstrate how biochemical and electrochemical activities vary by depth of biofilm and their dependence on electrode potential and electron transfer rate.
Fundamental research at the nano- and microscale to understand how biofilms interact with wounds in the presence of hyperosmotic agents (Department of Defense Congressionally Directed Medical Research Programs)
Biofilm-related wound infections acquired in the field or in field hospitals are a significant challenge for military preparedness. Both Acinetobacter and multi-resistant Staphylococcus aureus (MRSA) are primary agents of concern to military health care. If chronic wounds are not treated successfully it could lead to protracted antimicrobial therapies and serious problems including amputation and fatal sepsis. To avoid such problems, it is necessary to both kill bacteria and prevent formation of biofilms thereby converting a non-healing wound into a healing wound and improving outcomes and reducing healthcare costs. While preventing or controlling wound biofilms appears to be critical to successful recovery from battlefield wounds, little is known about biofilm structure and activity on wounds in the presence of hyperosmotic agents at the micro and nano-scales. Our goal is to investigate the fundamental mechanisms of wound-biofilm interactions in the presence of selected hyperosmotic agents using innovative nano- and microscale tools that have not been applied to wound biofilms before.
Depth-Resolved Nanospray Desorption Electrospray Ionization Mass Spectrometry in Biofilms
The goal of this reserach is to develop a mass spectrometry (MS) sensor which will allow us to obtain in situ, depth-resolved analyses of metabolites and possibly proteins. The project focuses on a demonstration of the technology, which can be applied to microbial fuel cells or subsurface biofilms used in heavy metal bioremediation.
Chemical gradients in Hot Lake microbial mat (DOE/PNNL)
The goal of this research is to determine chemical gradients in Hot Lake microbial mat in the laboratory and in the field using the microsensors and understand energy transformation mechanism.
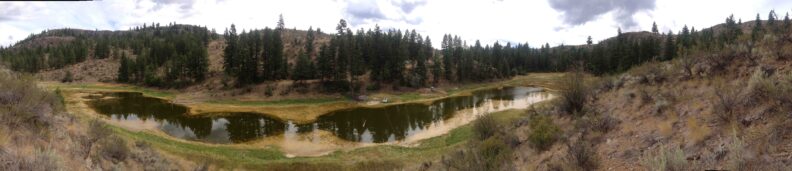
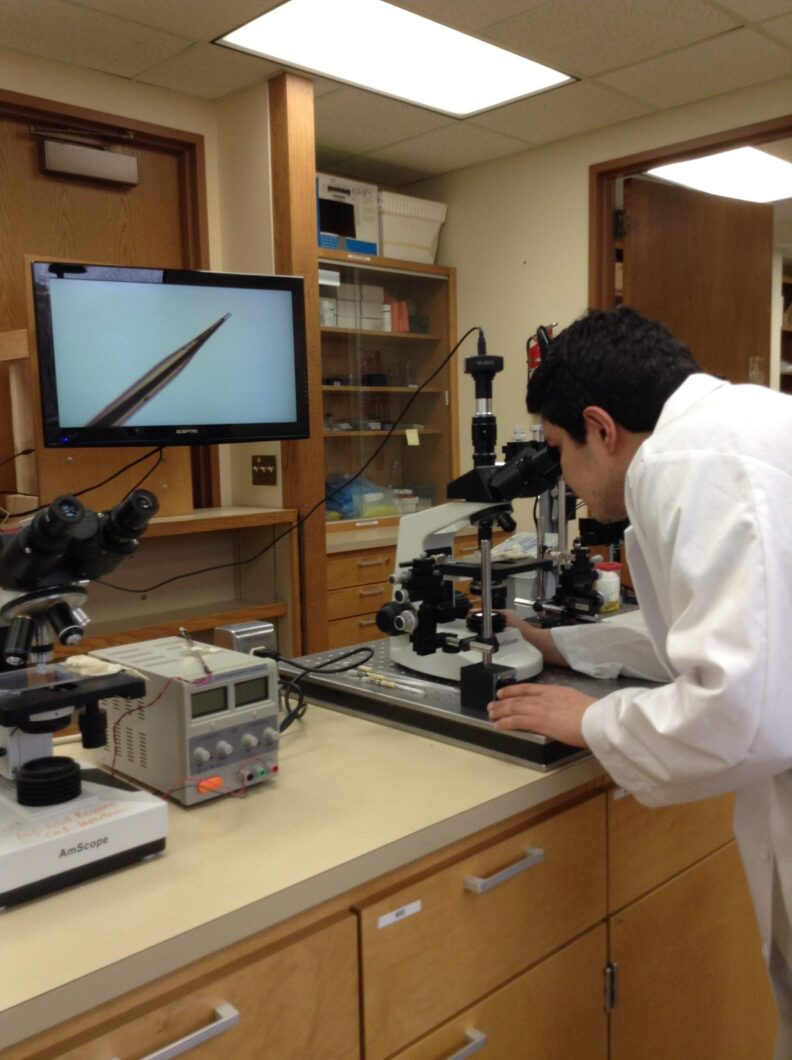
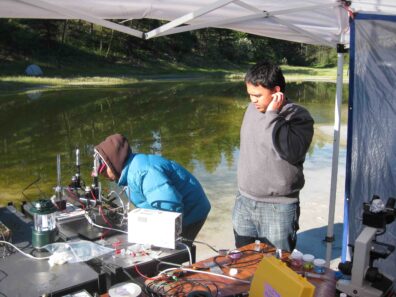
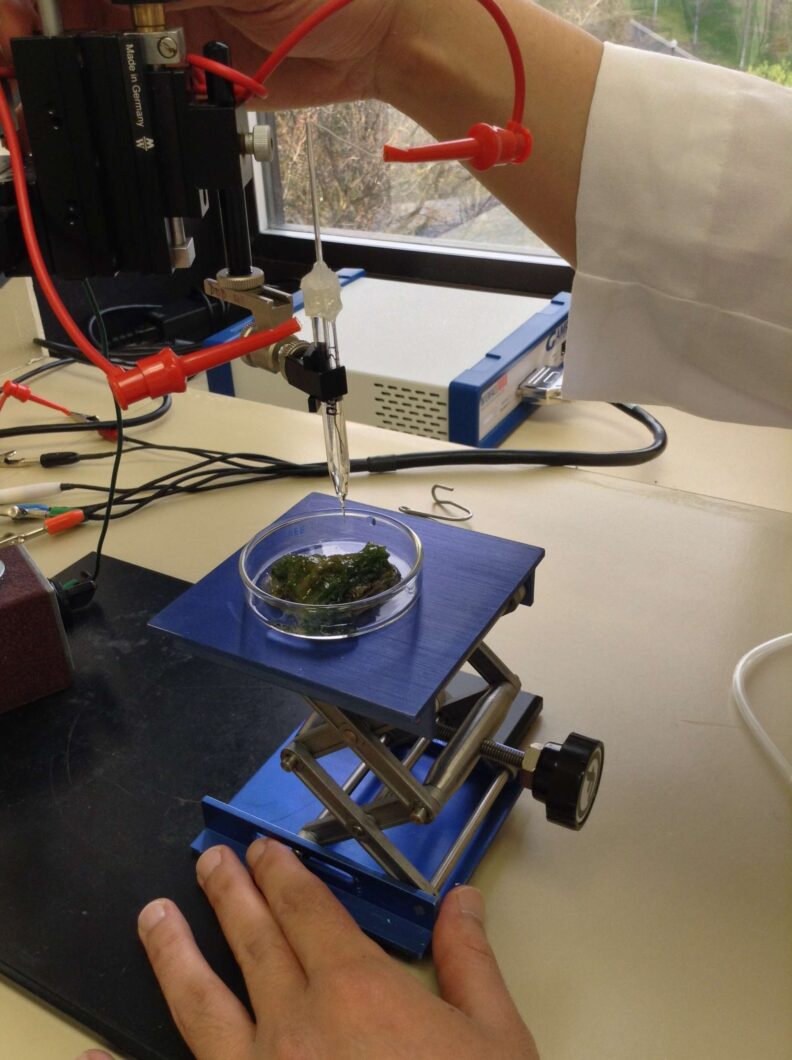
Microscale Metabolic, Redox and Abiotic Reactions in Hanford 300 Area Subsurface Sediments
Haluk Beyenal1 (PI), Jim Fredrickson2, Jeffrey S. McLean3, Bin Cao1,2, and Paul D. Majors2
1Washington State University, Pullman, WA; 2Pacific Northwest National Laboratory, Richland, WA;
3J. Craig Venter Institute, La Jolla, CA.
Collaborators: Kenneth M. Kemner, Bhoopesh Mishra, and Maxim I. Boyanov (Argonne National Laboratory, Argonne, IL); Matthew J Marshall, Liang Shi, David W Kennedy, Roslyn N. Brown, Yijia Xiong, Margaret F. Romine, Mary S. Lipton, Nancy G Isern (Pacific Northwest National Laboratory, Richland, WA)
The U.S. Department of Energy Hanford 300 Area (300A) site experiences periodic hydrologic influences from the nearby Columbia River as a result of changing river stage, which causes changes in groundwater elevation, flow direction and water chemistry. An important question is the extent to which the mixing of Columbia River water and groundwater impacts the speciation and mobility of uranium (U). This year, we designed experiments to mimic interactions among U, oxic groundwater or Columbia River water, and 300A sediments in the subsurface environment of Hanford 300A. The results revealed that U was immobilized by 300A sediments predominantly through reduction (80-85%) when the column reactor was fed with oxic, organic-amended synthetic groundwater (OA-SGW). The reduced U in the 300A sediments fed with OA-SGW was relatively resistant to remobilization by oxic Columbia River water. Oxic Columbia River water resulted in U remobilization (~7%) through desorption, and most of the U that remained in the 300A sediments fed with OA-SGW (~93%) was in the form of uraninite nanoparticles. These results revealed that: 1) the reductive immobilization of U through OA-SGW stimulation of indigenous 300A sediment microorganisms may be viable in the relatively oxic Hanford 300A subsurface environments and 2) with the intrusion of Columbia River water, desorption may be the primary process resulting in U remobilization from OA-SGW-stimulated 300A sediments at the subsurface of the Hanford 300A site.
In continuation of our work related to understanding how heavy metals interact with subsurface biofilms at the microscale, noninvasive nuclear magnetic resonance imaging (MRI) and spectroscopy (MRS) approaches were used to monitor spatiotemporal responses of live S. oneidensis MR-1 biofilms to U(VI) and Cr(VI). MRI and spatial mapping of diffusion in the biofilms revealed that, although the overall biomass distribution was not significantly altered upon exposure to U(VI) or Cr(VI), microenvironments in the biofilm matrix changed as indicated by localized changes (~ 20 microns) of water diffusivity in the biofilms, suggesting potentially important contaminant-induced changes in structural or hydrodynamic properties of biofilm matrix upon exposure to the metals. We also quantitatively demonstrate that the responses of cellular metabolism in biofilms interacting with environmental contaminants are spatially stratified, implying the possibility that the strategies for detoxification or adaptation utilized by cells in biofilms may be altered in response to the local microenvironments. Lastly, we developed a 2-dimensional mathematical model to predict substrate utilization and metabolite production rates in Shewanella oneidensis MR-1 biofilms as well as U immobilization by considering reduction and adsorption processes in the cells and in the extracellular polymeric substances (EPS). The model included the production of EPS using the experimental data we generated last year. The EPS bound to the cell surface and distributed in the biofilm were considered as bound EPS (bEPS) and loosely-associated EPS (laEPS), respectively. COMSOL® Multiphysics finite element analysis software was used to solve the model numerically. We used a custom designed biofilm reactor placed inside a nuclear magnetic resonance (NMR) micro-imaging and spectroscopy system, and monitored substrate utilization and metabolite production rates to compare model with experimental data.